How a quest for gold gave us sparkles in the sky.
Modern fireworks shows are truly spectacular. There are so many different colors, sounds, and shapes! But did you know that fireworks aren’t actually a modern invention? People have actually been using fireworks for big celebrations for over a thousand years. That special feeling of staring in awe at a fireworks display, a crowd of people around you all looking up into the same darkened sky, is one that has been shared by millions of people throughout history.
Table of Contents:
A Sparking Interest
Fireworks are believed to date all the way back to 200 BCE in China. People noticed that throwing pieces of bamboo into the fire would cause an explosion and a loud “bang”! These loud sounds were thought to scare away evil spirits.
The explosion itself was caused by the hollow segments inside of bamboo. When thrown into the fire, the air inside the bamboo quickly heats up and expands, loudly cracking the bamboo apart.
Alchemy and the Eternal Search
Before the field of chemistry existed, there was alchemy. Alchemists were inspired by myths and legends about an elixir of life, which could turn any metal into gold and increase the drinker’s lifespan. With the fantastical goal of creating this elixir, alchemists got to work systematically combining naturally occurring minerals and elements, observing their reactions, and hoping to strike gold. Although alchemists never succeeded in discovering the formula for the elixir of life (if they did, they’re very good at keeping it a secret!), many of the products of their experiments ended up being useful in other ways. Despite being motivated by such a wild goal, alchemists followed careful processes which laid the foundation for the Scientific Method.
So people knew how to make the “bang!” part of fireworks for a long time. But what about the rocket that shoots into the air, or the beautiful colors? Those didn’t come until many centuries later.
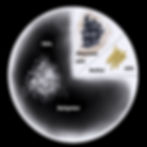
Around 900 CE, an alchemist mixed together saltpeter (a mineral that is now more commonly known by its chemical name: potassium nitrate), charcoal (the dark carbon pieces leftover after a fire), and sulfur (an element that smells like rotten eggs). The alchemist was probably trying to create a mythical substance that would give a person eternal life. Instead they created a highly flammable and explosive mixture: gunpowder. Now bamboo shoots or paper tubes could be filled with gunpowder and lit on fire to cause even more exciting sounds and lights!
And in the 1300s, Chinese scientists discovered a way to create colored smoke. By the 1600s, they were recording recipes for fires that produced various colors of smoke, and even one recipe for smoke with silver sparkles in it.
They also recorded recipes for yellow, green, and white flames. All of these reactions came from adding specific metallic compounds to the flames. For example, arsenical sulfide creates yellow flames, and copper acetate makes green.
Like countless other technologies that were shared across the world via the Silk Road, fireworks soon became widespread across Western and Central Asia and Europe. They became a popular part of major holidays and celebrations across the globe, though for the most part they were plain orange since no one had yet discovered how to add colors to these explosive rockets.
It wasn’t until the late 1700s that European chemists started to notice that adding pieces of certain metals could change the color of gunpowder sparks. Up to this point, changing the color of the sparks relied on finding the right compound in nature. But in the 1800s, there was a revolution: chemists began to figure out how to synthesize chemical compounds that were rare or didn’t occur in nature. This opened the door for bright and colorful fireworks as we know them!
Now in Technicolor
But why is it that adding these metallic salts to gunpowder produces color in the first place? This actually has to do with the atomic structure of the chemicals themselves. When the gunpowder ignites, it releases a ton of energy, some of which excites the electrons in the metal compounds. Soon after the energy is absorbed, the electrons release that energy in the form of light. The colors of light emitted by the electrons are called a “spectrum”. Every type of molecule or atom has a unique spectral “fingerprint” that is determined by the structure of its electrons.
Spectral "Fingerprints"
An atom or molecule’s unique spectral “fingerprint” is determined by the structure of its electrons. Since hydrogen is the simplest atom, with only one proton and one electron, let’s use it as an example. Usually the electron orbits very close to the proton. This is the lowest energy that it could have (and nature always prefers the lowest energy), so it’s called the “ground state” (n = 1). But there are also other “excited states” (n > 1) where the electron jumps to a different orbit. To get to the excited states, the electron needs an energy boost, but the boost must give it precisely the right amount of energy to get to the other level. Light can provide this boost!
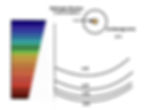
Light travels in little packets called photons, and each photon has a specific amount of energy. If a photon has exactly the right amount of energy that the electron needs to make the jump, then the electron will absorb the photon and jump to the excited state! There are many different combinations of energy states that an electron could jump between, and each jump requires its own amount of energy. So if you shine light onto a bunch of hydrogen, then each of these energy jumps will happen sometimes, and some light will come out of the other side of the hydrogen. But the leftover light will have some bands missing! This is called an absorption spectrum, since the missing bands were absorbed by the hydrogen to fuel its jump.
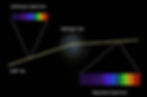
Eventually, the electron will let go of the extra energy it absorbed earlier. Remember, nature likes to be in the lowest energy state possible. And as the electron jumps back down to the ground state, it releases the energy once again in the form of a photon. The color of that light depends on the photon’s energy (which corresponds to the difference of energy levels that the electron jumped between). All these emitted photons make up the emission spectrum, and it is all the bands that were missing from the absorption spectrum.
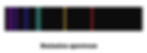
Both of these types of spectra are “fingerprints” for hydrogen– it is the only element with lines spaced exactly like this. Likewise, all atoms and molecules have their own unique spectral “fingerprints”, which allows scientists to decipher the exact composition of a gas. All they have to do is shine some light on it and see which colors are absorbed (absorption “fingerprint”), or look at the light that it is emitting (emission “fingerprint”).
Since we can identify chemical compounds by their spectral “fingerprints”, we can design the color of a firework by adding the chemicals that emit the light we want to see. If we want red fireworks, we’ll choose molecules that have red “fingerprints” like strontium. If we want blue, we need blue “fingerprints”, like copper. And so on for any color you could want!
Bang, Crackle, Whistle
The earliest form of fireworks - the pieces of bamboo thrown into fires - were really just noisemakers. To this day, a huge part of the fireworks experience is a “boom” so strong you can feel it shake your body. Fireworks might be known primarily for their color and appearance, but they wouldn’t be quite the same without the sounds they make.

The mixtures of gunpowder and metals change the color of the fireworks, but they also change the speed, energy, and ultimately the sound of the explosion. A fire needs three ingredients to burn: fuel, oxygen, and a spark. In the case of fireworks, the fuel is gunpowder (although it was discovered over 1000 years ago, it’s been tweaked and improved ever since). The addition of an oxidizer (a chemical compound that has several oxygen molecules attached, such as nitrate or chlorinate) provides oxygen to keep the fire going. And of course, lighting a firework’s fuse provides a spark to get the explosion going.
If all these ingredients are packed into a tiny space, then the fire will burn through all the fuel very quickly. As a result, the gasses from the reaction get hot and expand rapidly. This causes a huge “bang”!
On the other hand, small pieces of fuel could be scattered throughout the mix. Each piece of fuel will burn quickly, but in a much smaller region. These many, smaller explosions produce the softer, crackling types of fireworks.
How Do Whistles Work?
A whistling sound is the result of blowing air through a tube. But that doesn’t mean any plain tube will do; to produce a sound, you need a standing wave. Air travels in oscillating waves (meaning the air has peaks and troughs at regular, repeating intervals).
When these peaks and troughs line up with each other, then you’ve found a standing wave. A tube of a certain length can only fit specific wavelengths of these air oscillations, so the waves that fit are the standing waves for that tube.
Each standing wave has a frequency associated with it, and the motion of the air produced by these waves are what our ears perceive as sound! In fact, most sounds are described by their frequency. Depending on the shape of the tube and how the air is traveling through it, the tube will produce specific frequencies. This is how most instruments work. For example, by covering different holes on a recorder, you change the shape of the standing wave inside, resulting in a different tone.
Fireworks that make whistling sounds are specially formulated to burn their fuel so that the gasses released from the burning reaction create standing waves inside of their tube. This standing wave causes the whistle sound. Altering the shape of the tube or the angle of the exhaust vent produces varying sounds.
For Auld Lang Syne
Fireworks have been around for a looooong time. They’ve been an important part of celebrations and religious ceremonies across the globe and all year round. Alchemists accidentally created gunpowder (and consequently fireworks) during a failed attempt to make the elixir of life. But this failure actually ended up being a huge innovation! Gunpowder was very quickly weaponized in the form of rockets, bombs, and (of course) fuel for guns. This is an example of how curiosity and simple science experiments can have far reaching consequences. New scientific knowledge can be used by people to make things that are terrible, but also things that are beautiful.
A good fireworks show is fun and exciting to watch, with plenty of colors and sounds to keep our eyes and ears engaged. Paired with the smell of smoke and the feel of a particularly large “bang” resonating through your body, fireworks are truly a full sensory experience. But they also fit into a much bigger picture about the history of humans, our relationships with each other, and our fascination with the natural world. That makes a fireworks show all the more special to me!
Written by Madelyn Leembruggen
Edited by Ella King and Caroline Martin
Illustrations by Sachi Weerasooriya
Primary Sources and Additional Readings:
The Evolution of Fireworks by Alexis Stempien at The Smithsonian Science Education Center
The Chemistry of Fireworks: Bangs, Crackles & Whistles from Compound Interest
The Chemistry of Firework Colours from Compound Interest
Please don't build your own fireworks... but please do learn more about all the things that make fireworks special!
Investigate (30-45 minutes): Recreate Hydrogen's absorption spectrum as light from a nearby star passes through a cloud of Hydrogen gas.
Visualize (5-10 minutes): See sound with this sound wave generator! Adjust the frequency and amplitude of a sine wave to watch how the shape of a wave affects the sound our ears hear.
Build (1-1.5 hours): Fireworks launch into the sky using the power of rocket propulsion. Create a mini pop rocket to explore the basics of rocketry in your own backyard.